Table of Contents
- Introduction
- Cerebral Vasculature
- Hemodynamics
- Autoregulation of Cerebral Blood Flow
- Myogenic Control of Cerebral Blood Flow
- Metabolic Control of Cerebral Blood Flow
- Neurogenic Control of Cerebral Blood Flow
- Neurovascular Coupling
- Table 1: Different Physiological and Pathological conditions and their association with CBF auto-regulation
- Effect of Blood Gas Levels and Temperature
- Measurement of Cerebral Blood Flow
- Table 2: Common methods of cerebral blood flow measurement along with their features and limitations
- Further Readings
- Bibliography
Primary Category
Essential Neurology
P-Category
Secondary Category
Neurocritical Care
S-Category
Introduction
- Brain tissue has high metabolic demand and high neuronal activity requires functional hyperemia for supply of nutrients and Oxygen
- 20% of total body oxygen and 25% of glucose utilization is dedicated to brain
- Various autoregulatory mechanisms and local and neuronal factors work together to ensure adequate brain perfusion
- Cerebral blood flow is defined as the volume of arterial blood delivered to a unit mass of brain tissue per unit of time.
- It is described in ml of blood per 100 gram of brain tissue per minute.
- The normal value is 50 ml/100g/min
- White matter has relatively lower CBF i.e. 20 ml/100g/min
- The grey matter has relatively higher CBF i.e. 80 ml/100g/min
Cerebral Vasculature
- A highly complex interconnected network of vessels
- Feeding vessels include:
- Right and left internal carotid arteries anteriorly (~70 %)
- Right and left vertebral arteries posteriorly
- Vertebral arteries join to form the basilar artery
- Internal carotid and basilar artery are linked by the circle of Willis
- Circle of Willis connects these vessels on the inferior surface of the brain and consists of:
- Right and left anterior cerebral arteries (Branches of Internal Carotid Arteries)
- Anterior and posterior communicating arteries
- Middle cerebral arteries are also connected to the circle of Willis
Hemodynamics
- The flow of blood through the brain can be described by Ohm’s law:
Q = △P/R
Q is cerebral blood flow
△P is the pressure difference across the arterial and venous segment
R is resistance to flow
- This indicates:
- The direct relation between pressure difference and cerebral blood flow.
- Increase in pressure difference ➨ Increase in cerebral blood flow
- Decrease in resistance ➨ increase in cerebral blood flow
- Resistance of vessel is determined by the radius of vessel, length of the vessel, and hematocrit i.e viscosity (Poiseuille’s law)
- Cerebral blood flow is mainly determined by change in vessel radius especially in small vessels i.e arterioles
- To maintain adequate cerebral blood flow, a decrease in blood pressure will require an increase in vessel radius (opposite to normal vessel response).
Autoregulation of Cerebral Blood Flow
- Cerebral perfusion pressure (CPP) and cerebrovascular resistance (CVR) are main factors that control Cerebral blood flow.
CPP = Mean arterial pressure (MAP) - Intracranial Pressure (ICP)
MAP is average arterial pressure throughout one cardiac cycle
MAP = Diastolic Pressure + 1/3(Pulse Pressure)
ICP is the pressure of CSF in subarachnoid space
- Changes in CPP can be due to physiological (exercise) or pathological (TBI) processes.
- During cerebral autoregulation, optimal perfusion pressure is maintained by changes in CVR.
- Cerebral blood flow is kept relatively constant through the change in vessel diameter at the CPP range of 40-140 mmHg.
- CBF is regulated by the change in vessel radius between 50-150 mmHg MAP
- Cerebral autoregulation acts as a negative feedback mechanism to control the effect of increased MAP on CPP and keep CPP constant by reducing the radius of vessels when MAP is raised and vice versa.
- Static cerebral autoregulation ➨ CBF and MAP changes under steady-state conditions (minutes or hours)
- Dynamic cerebral autoregulation ➨ Transient changes in CBF and MAP and CBF (in seconds)
- The change in radius of vessels is the single most important factor in CBF autoregulation.
- Increased hematocrit ➨ increased viscosity ➨ low CBF
- Decreased hematocrit ➨ decreased viscosity ➨ increase CBF
- CBF is controlled by means of myogenic, metabolic, and neurogenic factors and partial pressure of arterial blood gases (CO2 and O2), cerebral metabolism, and the autonomic nervous system are primary determinants of CBF.
Figure 1: Cerebral Autoregulation
_(1).png&optimizer=image&quality=80&width=280)
Myogenic Control of Cerebral Blood Flow
- The myogenic mechanism is the response of small arteries to intravascular pressure changes
- Arterial membrane depolarization results in the influx of Ca2+ in the arterial wall through voltage-dependent Ca2+ channels.
- It is a fast process mediated by EDRF and NO and occurs within 1-10 sec of change in pressure.
Metabolic Control of Cerebral Blood Flow
- Metabolic response alteration in blood flow secondary to blood pressure changes
- Changes in diameter of cerebral vasculature in response to alterations in the concentration of vasodilator metabolites like adenosine
Neurogenic Control of Cerebral Blood Flow
- The neurogenic response consists of perivascular neurons that autoregulate the cerebral blood flow by gliovascular interactions and intramural vascular signaling.
- Astrocytes act as mediators for signaling from neurons to the cerebral vasculature.
- Extrinsic nerves from peripheral nerve ganglia control surface vessels (Pial arteries) and large arteries (ICA, VA, MCA) whereas intrinsic nerves from intrinsic brain neurons control vessels within the brain parenchyma which has high basal tone and respond less to neurotransmitters.
- The vessel tone of large arteries is maintained by:
- Sympathetic neurons ➨ Superior cervical ganglion ➨ Norepinephrine, Neuropeptide Y
- Parasympathetic neurons ➨ Otic and Pterygopalatine ganglion ➨ Acetylcholine, VIP, NO
- Capillary dilation is faster than arteriolar dilation and is assumed to be caused by the active relaxation of pericytes.
- Veins contain 3/4th of cerebral blood volume but contribute less to autoregulation due to their less baseline tone.
Neurovascular Coupling
- CBF is increased to accommodate greater metabolic demands of the brain via the process of neurovascular coupling.
- Neurovascular coupling is a temporal and regional linkage between neuronal activity and blood supply to the brain and is mediated by a number of metabolic by-products (lactate, adenosine), neurotransmitters (dopamine, acetylcholine), and vasoactive mediators (Ca2+, H+).
- Uncoupling due to any pathologic process results in loss of normal functioning of brain processes
Table 1: Different Physiological and Pathological conditions and their association with CBF auto-regulation
Different Physiological and Pathological conditions and their association with CBF autoregulation
Condition
Effect on CBF and Autoregulation
↓Baroreceptor sensitivity , ↓CBFV , CA unaffected
Progressive physical exercise → No effect on CA (↑HR, ↑ABP)
Hypoxic exercise → Impaired CA ( CBFV Maintained, ↓CO₂, ↑BBB Permeability)
Women with preeclampsia → Impaired CA
Acute Ischemic stroke (96 h) → dynamic CA impaired; static CA normal
CA worse in the first 5 days post-stroke and recovered over the following 5 months
Type 1 Diabetes: If autonomic neuropathy or diabetic ketoacidosis + → CA Impaired
Type 2 Diabetes: Distinct impairment in CA
Focal loss of static autoregulation: Impaired CA at CPP >95 mmHg and <55 mmHg
Neurovascular uncoupling with initial decrease in CBF, then intermediate period with normal or ↑CBF and again ↓CBF till recovery
Without Aura → CA maintained
With Aura → CA Impaired
Degree of stenosis and collateral circulation determines the level of CA impairment
Recanalization/Endarterectomy restore CA
CA impaired → Decreased CBF → Vasospasm → Delayed Cerebral Ischemia
[Disturbed CA in 5 days post-SAH → ↑ risk of DCI (21 days)]
Ictal Period → ↑ Cerebral Metabolism, ↑ CBF
Postictal Period → ↓Cerebral Metabolism, ↓CBF
Severe the OSAS → Greater the CA disturbance
↓ CBFV , ↓ SaO2
CA preserved ; CBF might decrease if ABP is reduced to normal in chronic hypertensive patient
Note: CA: Cerebral autoregulation, ABP: Arterial Blood Pressure, OSAS: Obstructive Sleep Apnea Syndrome
Effect of Blood Gas Levels and Temperature
- Increased arterial CO₂ dilates the cerebral vessels by changing extracellular pH and thus increasing blood flow.
- 1 mmHg increase in arterial PaCO₂ leads to 3-6 % elevation in CBF whereas 1 mmHg decrease in PaCO₂ reduce the CBF by 1-3%
- Hypoxia below 50 mmHg is potent vasodilator and thus increases cerebral blood flow.
- Cerebral metabolic rate decreases with hypothermia. 1 degree Celsius decrease in temperature reduces cerebral metabolic rate by 6-7 % and CBF decreases proportionately.
Figure 2: Relationship between PaCO₂ and PaO₂ and Cerebral Blood Flow
_(1).png&optimizer=image&quality=80&width=280)
Measurement of Cerebral Blood Flow
- The direct methods measure the delivery of arterial blood to the capillary bed and measure the amount of tracer delivered to brain tissue by blood flow.
- Most of the methods use exogenous or endogenous compounds whose passage is taken as an indicator of cerebral blood flow. These methods rely on Fick Principle.
- Indirect methods measure cerebral perfusion indirectly and do not quantify the direct measure of blood delivered to brain tissue.
Table 2: Common methods of cerebral blood flow measurement along with their features and limitations
Common methods of cerebral blood flow measurement along with their features and limitations
Methods
Features
Invasiveness
Limitations
Nitrous oxide Method
(Kety & Schmidt Method)
Invasive
Intravascular measurement so can’t be repeated multiple times
Xe-CT
Non-Invasive
Uncomfortable for patients
High radiation dose
limited spatial resolution
SPECT (Single photon emission computed tomography)
Minimally invasive
Expensive
Not quantitative
High radiation dose
Inaccurate for low CBF
PET (Positron emission tomography)
(Gold Standard)
Minimally invasive
Very-expensive
High radiation dose
ASL - MRI
(Arterial spin labeling - MRI)
Non-Invasive
Inaccurate for low and high CBF
Less accurate than PET
Low SNR
MRI - DSC
(Dynamic susceptibility contrast-MRI)
Minimally invasive
Limited number of measurements
Avoided in sensitive patients
TCD (Transcranial Doppler Ultrasound)
Non-Invasive
No volume estimation
Prone to personal error
Measure CBFV
Phase Contrast MRI
Non-invasive
Intravoxel phase dispersion
Displacement artifacts
NIRS (Near-Infrared Spectroscopy)
Non-invasive
Complexity of signal recorded
CT Perfusion
Minimally invasive
Not suitable for all patients
Accuracy is contrast dependent
Derived from: Cerebral blood flow and autoregulation: current measurement techniques and prospects for noninvasive optical methods. Cerebral Autoregulation: Control of Blood Flow in the Brain
Further Readings
Cerebral blood flow and autoregulation: current measurement techniques and prospects for noninvasive optical methods. Neurophotonics, 3(3), 031411.
Cerebral Blood Flow Measurements in Adults: A Review on the Effects of Dietary Factors and Exercise. Nutrients, 10(5), 530.
Bibliography
- Clarke DD, Sokoloff L. Circulation and energy metabolism of the brain. In: Basic Neurochemistry, Siegel G, Agrano BV, Albers RW, Molino PV (Eds.). New York: Raven Press, 1989: pp. 565–590
- Payne S. Cerebral autoregulation control of blood flow in the brain. Berlin, Germany: Springer; 2016
- Rudzinski W, Swiat M, Tomaszewski M, Krejza J. Cerebral hemodynamics and investigations of cerebral blood flow regulation. Nucl Med Rev Cent East Eur. 2007;10:29–42.
- Vavilala M. S., Lee L. A., Lam A. M., “Cerebral blood flow and vascular physiology,” Anesthesiol. Clin. N. Am. 20, 247–264 (2002).10.1016/S0889-8537(01)00012-8
- Berlin DA, Bakker J. Starling curves and central venous pressure. Crit Care. 2015 Feb 16;19:55.
- Cipolla MJ, Li R, Vitullo L. Perivascular innervation of penetrating brain parenchymal arterioles. J Cardiovasc Pharmacol 2004; 44: 1–8
- Paulson OB, Strandgaard S, Edvinsson L. Cerebral autoregulation. Cerebrovasc Brain Metab Rev. 1990 Summer;2(2):161-92. PMID: 2201348.
- Willie CK, Tzeng Y-C, Fisher JA, et al. Integrative regulation of human brain blood flow. J Physiol 2014. doi:10.1113/jphysiol.2013.268953
- Phillips AA, Ainslie PN, Krassioukov AV, et al. Regulation of cerebral blood flow after spinal cord injury. J Neurotrauma 2013; 30: 1551–1563
- Edvinsson L. Characterization of the contractile effect of neuropeptide Y in feline cerebral arteries. Acta Physiol Scand 1985; 125: 33–41
- Hamel E. Perivascular nerves and the regulation of cerebrovascular tone. J Appl Physiol 2006; 100: 1059–1064
- Girouard H., Iadecola C., “Neurovascular coupling in the normal brain and in hypertension, stroke, and Alzheimer disease,” J. Appl. Physiol. 100, 328–335 (2006).10.1152/japplphysiol.00966.2005
- Knot H. J., Nelson M. T., “Regulation of arterial diameter and wall [Ca2+] in cerebral arteries of rat by membrane potential and intravascular pressure,” J. Physiol. 508, 199–209 (1998).10.1111/j.1469-7793.1998.199br.x
- Kontos H. A., “Regulation of the cerebral circulation,” Ann. Rev. Physiol. 43, 397–407 (1981).10.1146/annurev.ph.43.030181.002145
- Iadecola C., “Neurovascular regulation in the normal brain and in Alzheimer’s disease,” Nat. Rev. Neurosci. 5, 347–360 (2004).10.1038/nrn1387
- Nippert, A. R., Biesecker, K. R., & Newman, E. A. (2018). Mechanisms Mediating Functional Hyperemia in the Brain. The Neuroscientist : a review journal bringing neurobiology, neurology and psychiatry, 24(1), 73–83. https://doi.org/10.1177/1073858417703033
- Phillips, A. A., Chan, F. H., Zheng, M. M., Krassioukov, A. V., & Ainslie, P. N. (2016). Neurovascular coupling in humans: Physiology, methodological advances and clinical implications. Journal of cerebral blood flow and metabolism : official journal of the International Society of Cerebral Blood Flow and Metabolism, 36(4), 647–664. https://doi.org/10.1177/0271678X15617954
- Cipolla MJ. The Cerebral Circulation. San Rafael (CA): Morgan & Claypool Life Sciences; 2009. Chapter 3, Perivascular Innervation. Available from: https://www.ncbi.nlm.nih.gov/books/NBK53087/
- E. Shimosegawa, J. Hatazawa, Hemodynamic and Metabolic Disturbances in Acute Cerebral Infarction, Editor(s): Arthur W. Toga, Brain Mapping, Academic Press, 2015, Pages 829-838, ISBN 9780123973160 https://doi.org/10.1016/B978-0-12-397025-1.00096-8
- Warnert EA, Murphy K, Hall JE, Wise RG (2015) Noninvasive assessment of arterial compliance of human cerebral arteries with short inversion time arterial spin labeling. J Cereb Blood Flow Metab 35(3):461–468
- Willie, C. K., MacLeod, D. B., Smith, K. J., Lewis, N. C., Foster, G. E., Ikeda, K., Hoiland, R. L., & Ainslie, P. N. (2015). The contribution of arterial blood gases in cerebral blood flow regulation and fuel utilization in man at high altitude. Journal of cerebral blood flow and metabolism : official journal of the International Society of Cerebral Blood Flow and Metabolism, 35(5), 873–881. https://doi.org/10.1038/jcbfm.2015.4
- Alifia Tameem, MBBS MD FRCA, Hari Krovvidi, MD FRCA, Cerebral physiology, Continuing Education in Anaesthesia Critical Care & Pain, Volume 13, Issue 4, August 2013, Pages 113–118, https://doi.org/10.1093/bjaceaccp/mkt001
- Payne, S. (2016). Cerebral Autoregulation: Control of Blood Flow in the Brain (SpringerBriefs in Bioengineering) (1st ed. 2016 ed.). Springer.
- Fantini, S., Sassaroli, A., Tgavalekos, K. T., & Kornbluth, J. (2016). Cerebral blood flow and autoregulation: current measurement techniques and prospects for noninvasive optical methods. Neurophotonics, 3(3), 031411. https://doi.org/10.1117/1.NPh.3.3.031411
- Butterworth JF, Mackey DC, Wasnick JD, Morgan and Mikhail's Clinical Anesthesiology, 5th ed. McGraw-Hill; 2013
AizaMD™: Revolutionizing Clinical Documentation
Discover the power of our ambient clinical documentation system, designed to transform clinical encounters into structured SOAP notes with unmatched ease. Experience exceptional value for less than $3 per day—cheaper than your daily coffee!
- Save Time: Free up over 90 minutes daily for each provider.
- Boost Revenue: Increase daily revenue by at least $1,000 per provider.
- Enhance Coding Quality: Our detailed documentation supports superior coding accuracy, ensuring optimal reimbursement.
- Maximize Engagement and Interaction: Dedicate more time to patient care and less to typing, fostering richer and more effective conversations between clinicians and patients
AizaMD™: Automated Radiology Report Generation!
Discover our breakthrough Radiology AI reporting platform built on Ambient AI. It enhances productivity and minimizes fatigue. Benefit from best-in-class accuracy with our automated radiology report generation, all at market-leading pricing.
📈 Efficiency: Cut dictation times by up to 50% (Less words, More report!
🎯 Focus: Keep your eyes on the images, not the keyboard!
💸 Revenue: Boost revenue by at least 20%
📑 Clarity: Patient summary in plain English
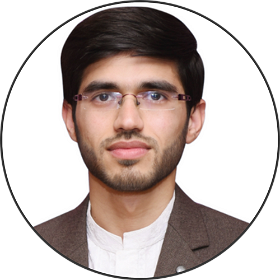
Written by
CSO at NeuroCare.AI, Postdoctoral Intern at Global Innervation, Anne Rowling Clinic Regenerative Neurology Scholar